Graphical Abstract
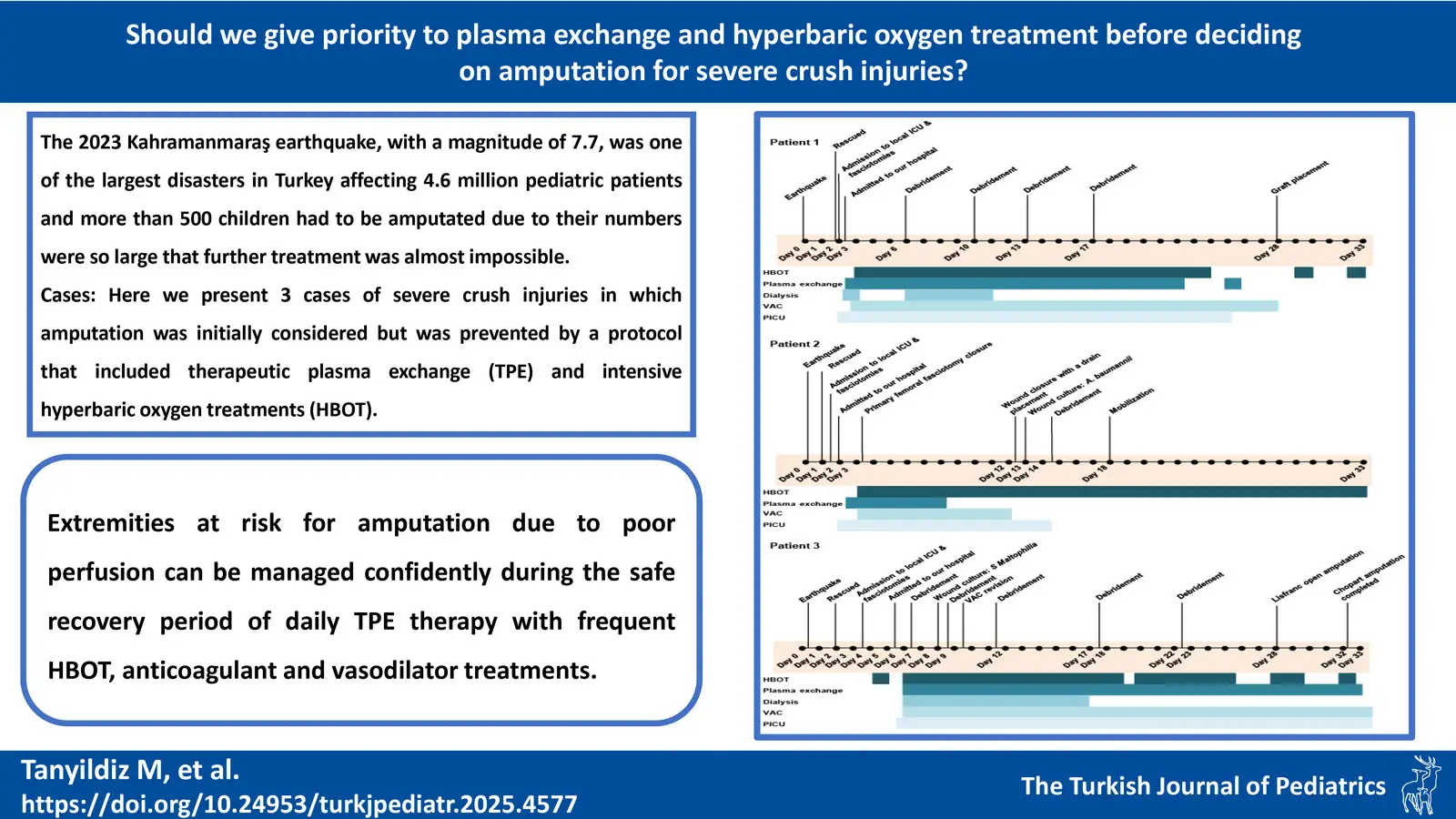
Abstract
Background. The most common medical sequelae after earthquakes are crush injuries and syndromes that require urgent and well-organized care, which further complicates the approach in the face of overstretched resources. The 2023 Kahramanmaraş earthquake, with a magnitude of 7.7, was one of the largest disasters in Türkiye, affecting 11 cities with a population of about 13.5 million people and claiming more than 50,000 deaths. Approximately 4.6 million pediatric patients were affected, with over 500 children undergoing amputations. The overwhelming number of cases rendered further treatment efforts nearly unfeasible.
Cases. Here we present three cases of severe crush injuries in which amputation was initially considered in both primary and our tertiary centers but was prevented by a protocol that included therapeutic plasma exchange (TPE) and intensive hyperbaric oxygen treatments (HBOT). Based on our review of the literature, this appears to be the first case series documenting the use of therapeutic plasma exchange (TPE) in the management of crush injury.
Conclusion. In extremities at risk for amputation, TPE therapy is crucial to preventing disseminated intravascular coagulation, systemic inflammatory response syndrome, and the accompanying multiorgan failure. It has been shown that extremities at risk for amputation due to poor perfusion can be managed confidently during the safe recovery period of daily TPE therapy with frequent HBOT, anticoagulant and vasodilator treatments, frequent wound care to prevent the development of infection, prophylactic antibiotics, vacuum-assisted closure therapy, and debridement when necessary.
Keywords: amputation, crush injury, hyperbaric oxygen treatment, therapeutic plasma exchange, pediatric protocol
Introduction
More and more medical professionals are being called upon to treat crush injuries and syndromes following human-made or natural disasters.1 These disasters can devastate numerous lives in an instant, significantly diminishing the affected individuals’ will to survive. The Kahramanmaraş earthquake of 2023 has been one of the clearest and most painful examples of this concept of the struggle for life. A magnitude of 7.7 was enough to destroy 11 cities in Türkiye, where approximately 13.5 million people lived.2
Numerous children underwent amputations as the possibility of further treatment was deemed unattainable. In this article, we aim to share the invaluable lessons learned by Turkish healthcare professionals through these challenging experiences, hoping they will serve as guidance. A team of healthcare professionals from our hospital was dispatched to the earthquake’s epicenter to provide care for pediatric victims. While they treated numerous patients on-site, three children were subsequently transferred to our hospital after achieving stable hemodynamics and metabolic balance. However, Doppler ultrasound evaluations revealed an absence of blood flow to the injured extremities in these cases. The aim of this article is to provide healthcare professionals with a pediatric protocol that includes therapeutic plasma exchange (TPE) and intensive hyperbaric oxygen treatments (HBOT) on how to manage these patients at high risk of amputation in both primary and tertiary care centers.
Additionally, hyperbaric oxygen therapy (HBOT) is documented in the literature to aid cellular respiration and prevent anaerobic infection in trauma cases, as well as reduce edema through increased extravascular fluid resorption.3,4 HBOT has also demonstrated effectiveness in severe limb injuries, where it supports wound healing and potentially mitigates the need for amputation in patients with high Mangled Extremity Severity Scores (MESS) (≥7).5 To our knowledge, this study represents the first cases series to investigate TPE in the context of crush injury.
Case Presentations
We present three cases of severe crush injuries in which amputation was initially considered but ultimately avoided through the implementation of a comprehensive treatment protocol that included TPE and intensive HBOT (Fig. 1).
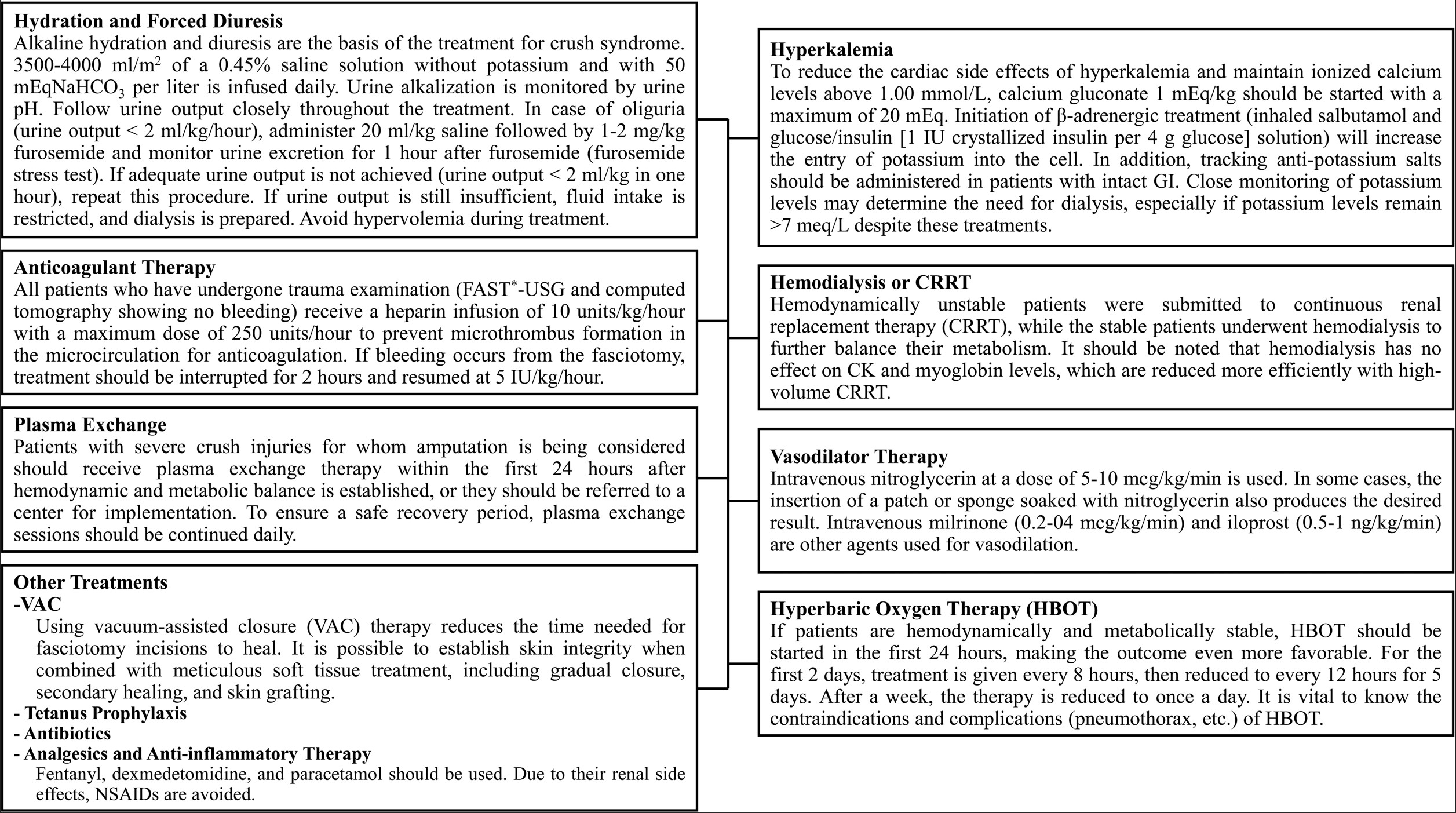
Patient-1
A 16-year-old girl was rescued 38 hours after the earthquake and presented with acute kidney injury and significant damages in the left lower limb, which was ecchymotic, edematous, and cold. The left leg had been subjected to crushing and compartment syndrome, and there were notable injuries, including an L1 vertebra fracture and a left fibula fracture. Three fasciotomy sites were noted, and Doppler ultrasonography revealed no detectable flow in the dorsal pedal artery. Initial recommendations by the orthopedic and trauma department included below-knee amputation. However, after implementing our treatment protocol on the 3rd day post-earthquake, the limb was salvaged through five debridement procedures, hemodialysis, 22 TPE sessions, and 33 HBOT sessions. The patient was discharged from the pediatric intensive care unit (PICU) after 24 days.
Patient-2
A 10-year-old boy was rescued 24 hours after the earthquake and admitted with an otherwise normal physical examination, except for three prior fasciotomy procedures on his left lateral gastrocnemius and both medial and lateral cruris. His left leg had also been subjected to crushing and compartment syndrome. He exhibited bullous lesions on his left foot, and his left toes were ecchymotic and anesthetic. The treatment protocol was initiated on the 2nd day after the earthquake. A week later, a Doppler ultrasound revealed a patent left dorsal pedal artery with biphasic-triphasic flow on spectral Doppler analysis, indicating significant improvement in vascular status. The patient underwent 3 debridement proceduress, 14 TPE sessions, and 45 HBOT sessions and was discharged after a 33-day PICU stay.
Patient-3
A 6-year-old male, was rescued 42 hours after the earthquake. He had undergone fasciotomies at a local PICU before being admitted to our facility. Upon admission, the left leg exhibited ecchymosis below the knee, with weak pulses in the left foot and an absent dorsal pedal pulse. The left toes showed signs of necrosis. The treatment protocol in our institution started on the 6th day after the earthquake. Doppler ultrasonography at admission indicated reduced peak systolic velocity in the left posterior tibial artery and no flow in the dorsal pedal and digital arteries. Initially, an above-knee amputation was considered. However, after following our treatment protocol, which included 3 debridement proceduress, 27 TPE sessions, and 30 HBOT sessions, the demarcation line previously suggested for below-knee amputation regressed to the dorsum of the foot, demonstrating significant improvement. On days 24 and 25, a Chopart amputation was performed.
Details of the interventions and other patient characteristics are outlined in Table I, Fig. 2 and Fig. 3. Written informed consent was obtained from the parents for this publication.
AKI: acute kidney injury, ALT: alanine transaminase, AST: aspartate transaminase, BMI: body mass index, BUN: blood urea nitrogen, DIC: disseminated intravascular coagulation, HBOT: hyperbaric oxygen therapy, HGB: hemoglobin, ICU: intensive care unit, LDH: lactate dehydrogenase, LYM: lymphocytes, MV: mechanical ventilation, NEU: neutrophils, PE: plasma exchange, PLT: platelets, VAC: vacuum-assisted closure, WBC: white blood cells. | |||
Table I. Demographic and clinical characteristics of the patients. | |||
Patient 1 | Patient 2 | Patient 3 | |
Age | 16 years 5 months | 10 years 4 months | 6 years 1 months |
Sex | Female | Male | Male |
Weight (kg) | 59 | 60 | 21 |
BMI (kg/m2) | 20.4 | 21.3 | 12.4 |
Rescue time | 38 hours | 24 hours | 42 hours |
The day of the start of treatment protocol | 3rd day after the earthquake | 2nd day after the earthquake | 6th day after the earthquake |
Extremities subjected to crushing | Left leg | Left leg | Left leg |
Compartment syndrome | + | + | + |
Fracture | L1 vertebra/left fibula | - | - |
Lung injury | Pneumomediastinum | - | - |
Liver injury | - | - | - |
AKI | + | - | - |
Local infection | - | Acinetobacter baumannii | Stenotrophomonas maltophilia |
Systemic infection | - | - | - |
DIC | - | - | - |
Echocardiogram | Normal | Normal | Normal |
Fasciotomy | + | + | + |
Amputation | - | - | The left tarsometatarsal joint. |
Number of debridement procedures | 5 | 3 | 5 |
Hemodialysis | + | - | - |
Hemofiltration | + | - | - |
Number of PE | 22 | 14 | 27 |
Number of HBOT | 33 | 45 | 30 |
VAC | + | + | + |
MV support | - | - | - |
Tetanus prophylaxis | Fully vaccinated | Vaccine +/Ig G+ | Fully vaccinated |
Length of stay in ICU | 24 days | 33 days | 31 days |
Patient outcome | Inpatient service | Inpatient servise | Inpatient servise |
Laboratory test results on admission | |||
HGB (g/dL) | 17.4 | 9.6 | 15.6 |
PLT (103/ µL) | 384 | 260 | 508 |
WBC (103/ µL) | 21.29 | 12,57 | 23.4 |
NEU (103/ µL) | 17.75 (83.4%) | 8.60 (68%) | 19.2 (82%) |
LYM (103/µL) | 1.46 (6.8%) | 2.9 (23%) | 2.0 (9%) |
BUN (mg/dL) | 22 | 11 | 27 |
Urea (mg/dL) | 47 | 24 | 58 |
Creatinine (mg/dL) | 1 | 0.4 | 0.4 |
Uric acid (mg/dL) | 6.8 | 5.6 | 9.1 |
Sodium (mmol/L) | 133 | 132 | 136 |
Potassium (mmol/L) | 5.4 | 4.3 | 5 |
Calcium (mg/dL) | 8.4 | 1.1 | 10.2 |
Phosphorus (mg/dL) | 7.2 | 2.9 | 5 |
Albumin (g/dL) | 26.8 | 23.9 | 4.5 |
AST (U/L) | 1910 | 565 | 285 |
ALT (U/L) | 695 | 264 | 131 |
LDH (U/L) | 4500 | 564 | >750 |
Amylase (U/L) | 19 | N/A | 24 |
Creatine kinase (U/L) | 134117 | 603 | >1000 |
Lipase (U/L) | 13 | 11 | 6 |
Total bilirubin (mg/dL) | 0.9 | 0.23 | 0.6 |
Direct bil. (mg/dL) | 0.2 | 0.16 | 0.2 |
Urine analysis | |||
pH | 6 | 7 | 9 |
Protein (mg/dL) | 100 | 30 | 0 |
Hemoglobin | +++ | - | - |
Color | dark brown | straw color | Straw color |
Density | 1037 | 1037 | 1009 |
Myoglobin (µg/L) | 80500 | 65 | 32 |
Laboratory test results of the last workup | |||
HGB (g/dL) | 10.5 | 9.7 | 7.7 |
PLT (103/ µL) | 326 | 440 | 420 |
WBC (103/ µL) | 4.97 | 7.64 | 6.72 |
NEU (103/ µL) | 2.7 (54%) | 3.9 (51%) | 3.6 (53%) |
LYM (103/µL) | 1.5 (30%) | 3 (39%) | 2.1 (31%) |
BUN (mg/dL) | 7 | 11 | 18 |
Urea (mg/dL) | 14 | 23 | 39 |
Creatinine (mg/dL) | 0.7 | 0.4 | 0.2 |
Uric acid (mg/dL) | 4 | 4.9 | 1.2 |
Sodium (mmol/L) | 140 | 138 | 140 |
Potassium (mmol/L) | 4 | 4.3 | 3.6 |
Calcium (mg/dL) | 9.8 | 10.3 | 9.6 |
Phosphorus (mg/dL) | 4.1 | 5.8 | 4.5 |
Albumin (g/dL) | 42.4 | 38.7 | 37.1 |
AST (U/L) | 35 | 27 | 30 |
ALT (U/L) | 31 | 30 | 25 |
LDH (U/L) | 209 | 250 | 212 |
Creatine kinase (U/L) | 101 | 154 | 63 |
Total bilirubin (mg/dL) | 0.33 | 0.3 | 0.23 |
Direct bilirubin (mg/dL) | 0.18 | 0.16 | 0.13 |
pH | 8 | 7 | 8 |
Protein (mg/dL) | 0 | 0 | 0 |
Color | Straw color | Straw color | Straw color |
Density | 1013 | 1014 | 1004 |
Myoglobin (µg/L) | < 8 | < 8 | < 8 |
Discussion
The first treatments given in primary centers to patients rescued from the earthquake zone with severe crush syndrome are intravenous (IV) alkaline hydration, forced diuresis, correction of electrolyte imbalances, assessment of urgent need for dialysis, and ensuring hemodynamic and metabolic stability.6
In our protocol, in addition to these treatments, heparin infusion was started (in patients who underwent trauma work-up and were not found to have hemorrhage in US and tomography) in order to prevent microthrombus formation in the extremities with severe crush injuries and decreased or absent blood flow in the Doppler. Treatment-related bleeding or new thrombi were not observed in any patient when the heparin doses specified in the protocol were used. Similarly, vasodilating treatments such as nitroglycerin (IV, topical), milrinone, and iloprost (in patients with decreased or absent arterial phase currents) were initiated to maintain microcirculation and increase perfusion in the ischemic ends of the extremities. Treatment-related complications and hypotension were not observed in any patients when used at the doses indicated in the protocol.
In the case of a stable patient with crush injuries, where the affected extremities present as ecchymotic, edematous, and ischemic with no detectable pulses or blood flow on Doppler ultrasound, the critical question arises: should amputation be performed promptly in the primary earthquake region, or should the patient be given the opportunity to recover from both the physical and emotional toll, provided their condition remains stable? This article will attempt to find answers to these questions. These extremities that remain ischemic with no blood flow can cause many serious systemic and life-threatening events such as thrombocytopenia associated multi-organ failure (TAMOF), systemic inflammatory response syndrome (SIRS), disseminated intravascular coagulation (DIC), sepsis, and gas gangrene.7 Continuing to treat these patients without amputation in the primary setting is a very daring decision given the high volume of patients, as there is a risk of patient loss due to potential systemic complications. Even transferring the patient in this condition to a tertiary center may pose a great risk in terms of systemic complications that may develop along the way. At this point, TPE therapy provided a very safe recovery period, allowing both safe transfer of the patient to a tertiary center and the possibility of performing limb-preserving surgery with complete recovery or elimination of the demarcation line through more frequent prolonged use of HBOT in tertiary centers.
In recent years, extensive experience has been gained with the utility of TPE therapy in the treatment of sepsis, TAMOF, and DIC.8,9 The experience with TPE in crush injury has not yet been reported. Considering the pathophysiology of the event, crush injury leads to severe endothelial damage.7 This endothelial damage may trigger the inflammatory pathway, leading to the formation of a cytokine storm and the development of SIRS.7 The developing endothelial damage may also trigger the microthrombotic pathway, leading to excessive endothelial exocytosis and consequent release of large amounts of ultralarge von Willebrand factor (uLVWF) into the circulation.7 The enzyme ADAMTS13 is required to degrade the uVWF protein.7 If there is not enough ADAMTS13 enzyme to degrade the uLVWF, which enters the bloodstream in large quantities, the uLVWFs attach to the endothelium in the microcirculation, leading to the formation of microthrombi with the accumulation of platelet aggregates on them and compromising organ perfusion.7 Multiple organ failure occurs when other organs are also affected. The usual treatment is TPE when these two pathways are activated. Plasma exchange ensures both a decline in proinflammatory mediators of the inflammatory pathway and also clear and refreshing the elements of microtrombotic pathway.9 The timing of initiating treatment is critical to see the beneficial effect of TPE. Patient-1 who spent similar period under the debris and had even more severe crush injuries than Patient-3, did not need amputation due to early initiating TPE and the protocol, whereas partial amputation was needed in Patient-3 (Fig. 2).
The rationale for utilizing TPE in our patient cohort stems from the potential to mitigate the sequelae of severe crush injuries, including SIRS, DIC, and TAMOF. While our patients exhibited clinical stability, some presented with concerning signs indicative of potential extremity compromise, such as coldness, ischemia, and Doppler-confirmed absent blood flow. However, we refrained from immediate amputation, opting instead for comprehensive management in tertiary care facilities equipped to monitor these patients closely.
The implementation of TPE was particularly critical in the high-risk, post-earthquake environment, where the increased patient load posed significant challenges to effective monitoring for systemic complications. Prophylactic use of TPE aimed to prevent the onset of SIRS, DIC, and TAMOF, allowing time for effective interventions like HBOT, which was also employed early in treatment protocols.
Despite the absence of overt clinical manifestations of SIRS, DIC, or TAMOF in many patients, TPE therapy was maintained for approximately one month. This prolonged intervention was justified by the need to prevent potential complications associated with the underlying pathophysiological processes of crush syndrome, which include significant endothelial injury and inflammatory responses leading to microthrombi formation. The benefits of TPE in clearing inflammatory mediators and pro-thrombotic elements underscore its role in facilitating recovery and possibly enhancing outcomes when combined with other treatments.
Additionally, we observed that the trend of acute phase reactants in these patients could serve as an important indicator of inflammatory status and response to therapy (Fig. 3). Future analyses could provide insights into how different biomarkers correlate with clinical outcomes, thus reinforcing the rationale for the ongoing use of TPE in this patient population.
Furthermore, our experience aligns with literature suggesting the efficacy of TPE in similar scenarios, albeit less explored in crush injuries specifically. For instance, existing studies have illustrated the benefits of TPE in severe burn injuries, where similar pathophysiological (endothelial injury) mechanisms are at play.10 By addressing the cytokine storm and mitigating microthrombi formation through early intervention, TPE has demonstrated potential to avert drastic outcomes, such as limb amputation, as exemplified by our patients.
HBOT increases not only the dissolved oxygen partial pressure in the blood, but also ensures mitochondrial oxygenation.11 This allows aerobic respiration to continue in all cells and keeps tissues alive.11,12 HBOT has an anti-edematous effect by lowering capillary blood pressure and increasing extravascular fluid reabsorption.12 Since it keeps aerobic metabolism alive, it has an antibacterial effect by preventing the development of anaerobic organisms.5,12 One of its most important effects is the acceleration of wound healing by stimulating collagen synthesis and fibroblast migration.5,12 In our protocol, HBOT was administered to patients with extremities initially considered for amputation. The treatment schedule included sessions every 8 hours for the first two days, every 12 hours for the subsequent five days, and then once daily thereafter. Each treatment provided in monoplace hyperbaric chambers a pressure of 2.4 atmospheres absolute (ATA) for 90-120 minutes with compression and decompression periods.
We had one patient who was on dialysis due to acute kidney injury. We think that by choosing the high-volume continuous renal replacement therapy method in addition to TPE therapy in the first two days, it also contributed to cytokine clearance.
Using vacuum-assisted closure (VAC) for fasciotomies was one of the treatment’s most crucial components. It has been demonstrated that VAC therapy reduces the time needed for fasciotomy incisions to heal.13 Meanwhile, it should be remembered that a healed wound is merely the first stage of salvaging. Long-term rehabilitation procedures and other interventions will be required for a functional limb.
Patients at risk of amputation should be referred to tertiary care centers in a coordinated manner following metabolic and hemodynamic stabilization at primary centers, which experience high patient volumes in the aftermath of an earthquake. TPE plays a crucial role in preventing DIC, SIRS, and associated multiorgan failure in extremities at risk of amputation.
Conclusion
It has been shown that extremities at risk for amputation due to poor perfusion can be managed confidently during the safe recovery period of daily TPE therapy with frequent HBOT, anticoagulant and vasodilator treatments, frequent wound care to prevent the development of infection, prophylactic antibiotics, VAC therapy, and debridement when necessary.
In conclusion, our approach underscores the importance of a multifaceted treatment protocol that incorporates TPE, HBOT, and supportive measures to optimize patient outcomes in the face of severe crush injuries. Early intervention and close monitoring in specialized settings are vital to navigating the complexities associated with these critical conditions.
Ethical approval
Written informed consent was obtained from the parents for this publication.
Source of funding
The authors declare the study received no funding.
Conflict of interest
The authors declare that there is no conflict of interest.
References
- Sever MS, Erek E, Vanholder R, et al. The Marmara earthquake: epidemiological analysis of the victims with nephrological problems. Kidney Int 2001; 60: 1114-1123. https://doi.org/10.1046/j.1523-1755.2001.0600031114.x
- Turan Rİ. Magnitude 5 earthquake jolts Türkiye’s southern province. Anadolu Agency. March 3, 2023. Available at: https://www.aa.com.tr/en/turkiye/magnitude-5-earthquake-jolts-turkiye-s-southern-kahramanmaras-province/2835933 (Accessed on March 21, 2023).
- Gill AL, Bell CN. Hyperbaric oxygen: its uses, mechanisms of action and outcomes. QJM 2004; 97: 385-395. https://doi.org/10.1093/qjmed/hch074
- Cannellotto M, Yasells García A, Landa MS. Hyperoxia: effective mechanism of hyperbaric treatment at mild-pressure. Int J Mol Sci 2024; 25: 777. https://doi.org/10.3390/ijms25020777
- Jirangkul P, Baisopon S, Pandaeng D, Srisawat P. Hyperbaric oxygen adjuvant therapy in severe mangled extremities. Injury 2021; 52: 3511-3515. https://doi.org/10.1016/j.injury.2021.06.033
- Gibney RTN, Sever MS, Vanholder RC. Disaster nephrology: crush injury and beyond. Kidney Int 2014; 85: 1049-1057. https://doi.org/10.1038/ki.2013.392
- Chang JC. Sepsis and septic shock: endothelial molecular pathogenesis associated with vascular microthrombotic disease. Thromb J 2019; 17: 10. https://doi.org/10.1186/s12959-019-0198-4
- Putzu A, Schorer R, Lopez-Delgado JC, Cassina T, Landoni G. Blood purification and mortality in sepsis and septic shock: a systematic review and meta-analysis of randomized trials. Anesthesiology 2019; 131: 580-593. https://doi.org/10.1097/ALN.0000000000002820
- Knaup H, Stahl K, Schmidt BMW, et al. Early therapeutic plasma exchange in septic shock: a prospective open-label nonrandomized pilot study focusing on safety, hemodynamics, vascular barrier function, and biologic markers. Crit Care 2018; 22: 285. https://doi.org/10.1186/s13054-018-2220-9
- Klein MB, Edwards JA, Kramer CB, Nester T, Heimbach DM, Gibran NS. The beneficial effects of plasma exchange after severe burn injury. J Burn Care Res 2009; 30: 243-248. https://doi.org/10.1097/BCR.0b013e318198a30d
- Schottlender N, Gottfried I, Ashery U. Hyperbaric oxygen treatment: effects on mitochondrial function and oxidative stress. Biomolecules 2021; 11: 1827. https://doi.org/10.3390/biom11121827
- Millar IL, McGinnes RA, Williamson O, et al. Hyperbaric Oxygen in Lower Limb Trauma (HOLLT); protocol for a randomised controlled trial. BMJ Open 2015; 5: e008381. https://doi.org/10.1136/bmjopen-2015-008381
- Zannis J, Angobaldo J, Marks M, et al. Comparison of fasciotomy wound closures using traditional dressing changes and the vacuum-assisted closure device. Ann Plast Surg 2009; 62: 407-409. https://doi.org/10.1097/SAP.0b013e3181881b29
Copyright and license
Copyright © 2025 The Author(s). This is an open access article distributed under the Creative Commons Attribution License (CC BY), which permits unrestricted use, distribution, and reproduction in any medium or format, provided the original work is properly cited.